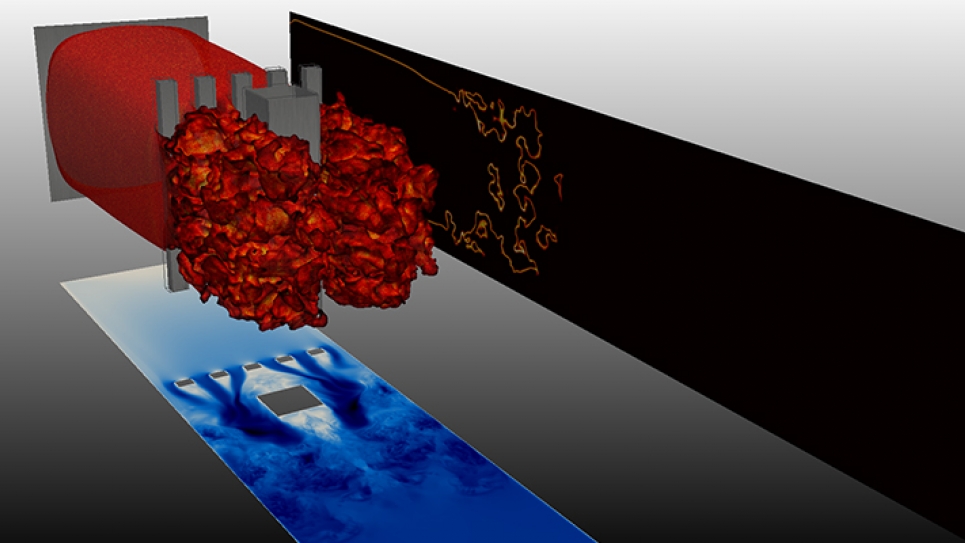
We have all heard similar news stories. What usually starts as a small fire quickly gets out of control and a house is engulfed in flame. Evidence often points to a stove, a space heater, or a forgotten cigarette as the culprit. Now take an industrial setting, throw into the mix any number of chemicals or gases and some form of oxidizer, and you have an even greater recipe for disaster.
These devastating effects occur as fire in an enclosed space begins to propagate, or spread, and heats the surrounding environment, creating a buildup of pressure. This overpressure can build very rapidly and explode in dramatic fashion, unless there is an opportunity for that pressure to be released.
Researcher Thierry Poinsot, from the European Centre for Research and Advanced Training in Scientific Computation (CERFACS), is leading an international effort to better understand the mechanics of flame and explosion propagation, from the early laminar flame phase to the more aggressive turbulent flame that can incite explosion. Computer resources from the Argonne Leadership Computing Facility (ALCF) are assisting researchers in the development of high fidelity tools to simulate realistic explosion scenarios.
“Currently, all the systems around us are certified for explosion risks using software that is totally ad hoc, based on correlations and not on real precise simulations. These certifications control the safety rules for buildings. If they are wrong, you can kill people,” says Poinsot.
This research, he believes, will lead to the employment of better safety mechanisms and regulations, both in buildings and offshore oil platforms. For instance, French oil and gas company, Total, is interested in Poinsot’s work as it applies to flame spread on offshore platforms in an effort to avoid catastrophes, such as the 2010 Deepwater Horizon explosion in the Gulf of Mexico that left 11 dead and many others injured.
In experiments conducted in Australia, dedicated chambers were outfitted with baffles and columns to better represent obstacles in an enclosed space and an explosion was produced using a premixed flame mixture of propane and air. The results, derived from a small laboratory explosion in a vessel of 0.25 m, were so interesting that the experimental setup was replicated in Norway at two larger scales: 1.5 and 6 m.
The explosions were monitored using such advanced techniques as particle image velocimetry, which tracks how minute particles introduced into the scenario move with the flame front. In all cases, the researchers looked at the propagation speed of the flame, which starts at 40 cm/s and ends at an astounding 250 m/s. They also monitor the pressure in the vessel that controls the spread of the flame. If the pressure is too large, the vessel explodes.
Working with the ALCF, Poinsot and his team used this data to validate the first-ever large eddy simulation (LES) of a building explosion. The simulation played out on a one-billion-cell mesh, or grid, and required the massive computing power of the ALCF’s IBM Blue Gene/Q supercomputer, Mira.
To ensure the simulations captured all phases of the flame development, the researchers began with the smaller 0.25 m experimental setup for LES validation. Originally conducted on a 20-million-cell grid, the Australian simulation was later recreated using one billion cells to determine whether the larger grid would still yield the same results.
The success of this operation was essential in upscaling to the Norway simulations, which operated on scales 36 and 600 times more difficult for the small and large scale experiments, respectively. These simulations were also carried out with one billion cells.
According to Poinsot, the Norway models captured the experimental results reasonably well, taking researchers one step closer to recreating real systems, though computational scientists at CERFACS and ALCF are working together to optimize resolution at these larger scales.
Through these computer simulations, researchers have begun to develop the world’s largest database on turbulent flames to calculate peak pressures in an enclosed environment given a certain geometry and chemical composition of a flame front. This information will guide designers and engineers toward the design of safer buildings and mechanisms that could potentially arrest the propagation of a flame.
“While we need one billion cell points for a 6 meter building, it shows that we can do it today with systems currently available to us. This suggests that we have a truly predictive method for full buildings, instead of relying on approximate—and dangerous—methods,” says Poinsot.
“This is not a crazy idea any more. And with larger machines, we can make it work in practice for real systems, for full buildings.”
This project was awarded computing time at the ALCF through DOE’s Innovative and Novel Computational Impact on Theory and Experiment (INCITE) program.