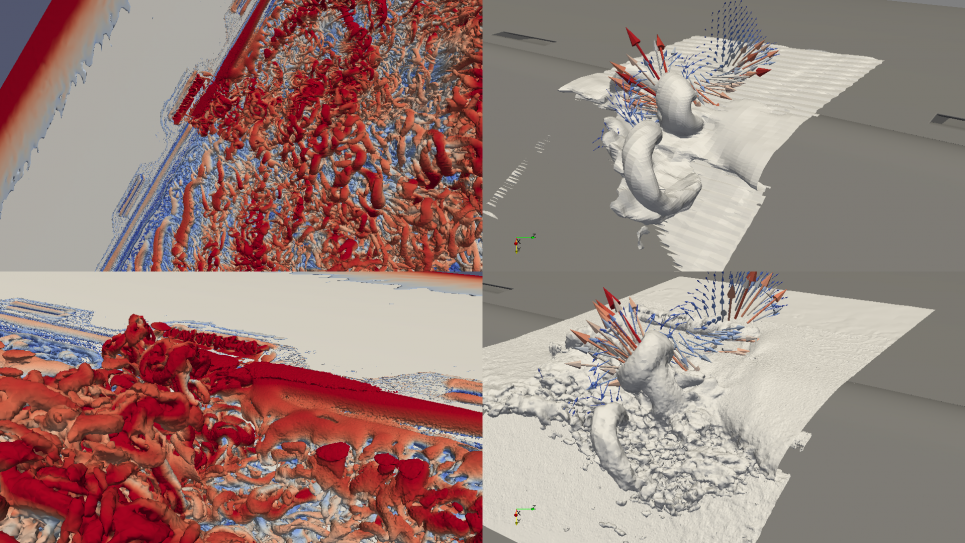
This project seeks to advance simulations in two complex flow regimes— aerodynamic flow control and multiphase flow—by pairing a computational fluid dynamics solver with anisotropic adaptation so as to solve solve diverse problems in aerodynamic flow control and nuclear reactor heat exchangers. Both applications stand to dramatically extend the state-of-the art in turbulent flow simulations.
This project seeks to advance simulations in two complex flow regimes: aerodynamic flow control and multiphase flow. Both simulations will exploit anisotropic adaptive unstructured grids to match mesh length scales precisely to solution requirements which, together with a solver that has already scaled to more than 3 Million (M) processes, will allow a dramatic advance in computational modeling and associated scientific and engineering insight.
The aerodynamic flow control task builds upon previous ESP and INCITE efforts where computational models of a vertical tail and rudder assembly with 12 synthetic jets were validated against experiments (predicting the same 20 percent increase in side force and same phase averaged jet structures) at a Reynolds number of 3.5e5. This Reynolds number is 53 times smaller than flight conditions. Herein, we propose to use Aurora to perform a series of simulations up to full flight conditions. This battery of simulations will be the first ever flight Reynolds number Detached Eddy Simulation of a full scale aerodynamic component, a vertical tail-rudder assembly. The vertical tail is sized to handle an engine-out condition which requires it to be much larger than what is needed for all other conditions in the flight envelope. The economic impact is directly related to the size of the stabilizer since it is a significant contributor to drag in cruise where much of the fuel is expended. If flow control can achieve the same side force with a 25 percent smaller vertical tail, the jet fuel usage could be reduced by 0.5 percent which would result in 300 million dollars per year savings at current prices.
The multiphase flow task will extend existing direct numerical simulations of bubbly flows within realistic nuclear reactor geometries to unprecedented scale; matching all flow conditions to reactor scale. The approach uses the combined level-set volume of fluid method to accurately track the liquid-vapor phase boundary and thus captures the detailed physics that is currently beyond phase- field modeling capability. The impact of the simulations will be fundamental insight into the physics of multi-phase flow which is critical to thermal management within nuclear reactor safety/accident modeling. The proposed simulations will provide a critical step forward in the improvement of safety margin predictions for existing light water reactors and facilitate the development of next generation energy systems, impacting both the safety and the cost of nuclear energy production.